Why the universe shouldn't exist at all
Why the universe shouldn't exist at all
By Don Lincoln
Updated 9:56 PM ET, Sat March 31, 2018
Don Lincoln, a senior physicist at Fermilab, does research using the Large Hadron Collider. He is the author of "The Large Hadron Collider: The Extraordinary Story of the Higgs Boson and Other Stuff That Will Blow Your Mind," and produces a series of science education videos. Follow him on Facebook. The opinions expressed in this commentary are his.
(CNN)"Why is there something, rather than nothing?" could be the oldest and deepest question in all of metaphysics. Long exclusively the province of philosophy, in recent years this question has become one that can be addressed by scientific methods. What's more, a new scientific advance has made it more likely that we will finally be able to answer this cosmic conundrum. This is a big deal, because the simplest scientific answer to that question is "We shouldn't exist at all."
Obviously, we know that there must be something, because we're here. If there were nothing, we couldn't ask the question. But why? Why is there something? Why is the universe not a featureless void? Why does our universe have matter and not only energy? It might seem surprising, but given our current theories and measurements, science cannot answer those questions.
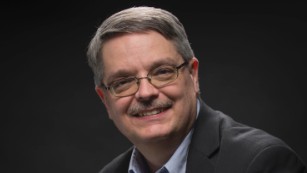
Don Lincoln
However, give some scientists 65 pounds of a rare isotope of germanium, cool it to temperatures cold enough to liquify air, and place their equipment nearly a mile underground in an abandoned gold mine, and you'll have the beginnings of an answer. Their project is called the Majorana Demonstrator and it is located at the Sanford Underground Research Facility, near Lead, South Dakota.
To grasp why science has trouble explaining why matter exists — and to understand the scientific achievement of Majorana -- we must first know a few simple things. First, our universe is made exclusively of matter; you, me, the Earth, even distant galaxies. All of it is matter.
However, our best theory for explaining the behavior of the matter and energy of the universe contradicts the realities that we observe in the universe all around us. This theory, called the Standard Model, says that the matter of the universe should be accompanied by an identical amount of antimatter, which, as its name suggests, is a substance antagonistic to matter. Combine equal amounts of matter and antimatter and it will convert into energy.
And the street goes both ways: Enough energy can convert into matter and antimatter. (Fun fact: Combining a paper clip's worth of matter and antimatter will result in the same energy released in the atomic explosion at Hiroshima. Don't worry though; since antimatter's discovery in 1931, we have only been able to isolate enough of it to make about 10 pots of coffee.)
An enigma about the relative amounts of matter and antimatter in the universe arises when we think about how the universe came to be. Modern cosmology says the universe began in an unimaginable Big Bang — an explosion of energy. In this theory, equal amounts of matter and antimatter should have resulted.
So how is our universe made exclusively of matter? Where did the antimatter go?
The simplest answer is that we don't know. In fact, it remains one of the biggest unanswered problems of modern physics.
Just because the question of missing antimatter is unanswered doesn't mean that scientists are completely clueless. Beginning in 1964 and continuing through to the present day, physicists have studied the problem and we have found out that early in the universe there was a slight asymmetry in the laws of nature that treated matter and antimatter differently.
Very approximately, for every billion antimatter subatomic particles that were made in the Big Bang, there were a billion-and-one matter particles. The billion matter and antimatter particles were annihilated, leaving the small amount of leftover matter (the "one") that went on to make up the universe we see around us. This is accepted science.
However, we don't know the process whereby the asymmetry in the laws of the universe arose. One possible explanation revolves around a class of subatomic particles called leptons.
The most well-known of the leptons is the familiar electron, found around atoms. However, a less known lepton is called the neutrino. Neutrinos are emitted in a particular kind of nuclear radiation, called beta decay. Beta decay occurs when a neutron in an atom decays into a proton, an electron, and a neutrino.
Neutrinos are fascinating particles. They interact extremely weakly; a steady barrage of neutrinos from the nuclear reactions in the sun pass through the entire Earth essentially without interacting. Because they interact so little, they are very difficult to detect and study. And that means that there are properties of neutrinos that we still don't understand.
Still a mystery to scientists is whether there is a difference between neutrino matter and neutrino antimatter. While we know that both exist, we don't know if they are different subatomic particles or if they are the same thing. That's a heavy thought, so perhaps an analogy will help.
Imagine you have a set of twins, with each twin standing in for the matter and antimatter neutrinos. If the twins are fraternal, you can tell them apart, but if they are identical, you can't. Essentially, we don't know which kind of twins the neutrino matter/antimatter pair are.
If neutrinos are their own antimatter particle, it would be an enormous clue in the mystery of the missing antimatter. So, naturally, scientists are working to figure this out.
The way they do that is to look first for a very rare form of beta decay, called double beta decay. That's when two neutrons in the nucleus of an atom simultaneously decay. In this process, two neutrinos are emitted. Scientists have observed this kind of decay.
However, if neutrinos are their own antiparticle, an even rarer thing can occur called "neutrinoless double beta decay." In this process, the neutrinos are absorbed before they get outside of the nucleus. In this case, no neutrinos are emitted. This process has not been observed and this is what scientists are looking for. The observation of a single, unambiguous neutrinoless double beta decay would show that matter and antimatter neutrinos were the same.
If indeed neutrinoless double beta decay exists, it's very hard to detect and it's important that scientists can discriminate between the many types of radioactive decay that mimic that of a neutrino. This requires the design and construction of very precise detectors.
So that's what the Majorana Demonstrator scientists achieved. They developed the technology necessary to make this very difficult differentiation. This demonstration paints a way forward for a follow-up experiment that can, once and for all, answer the question of whether matter and antimatter neutrinos are the same or different. And, with that information in hand, it might be possible to understand why our universe is made only of matter.
For millennia, introspective thinkers have pondered the great questions of existence. Why are we here? Why is the universe the way it is? Do things have to be this way? With this advance, scientists have taken a step forward in answering these timeless questions.
No comments:
Post a Comment